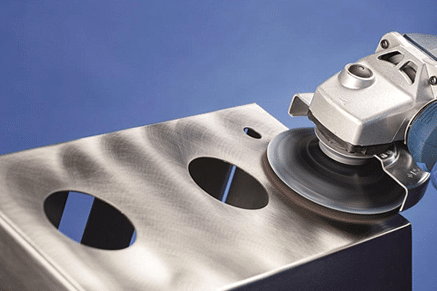
Table of Contents
What is Thermoplastic Injection Molding?
Thermoplastic injection molding is a transformative process where thermoplastic polymers are melted, injected into a mold, and cooled to form a solid shape. This method is pivotal in producing a myriad of plastic parts, from simple household items to complex automotive components.
Why are Thermoplastics Used in Injection Molding?
Thermoplastics, known for their pliability upon heating and solidity when cooled, are ideal materials for injection molding due to their recyclability and versatility in forming complex shapes and designs. Their adaptability makes them a preferred choice in various industries.
How Does Thermoplastic Injection Molding Work?
Thermoplastic injection molding, a cornerstone in modern manufacturing, involves transforming thermoplastic polymers into various plastic parts through a meticulous and controlled process. This technique is essential in producing items ranging from automotive components to consumer products, epitomizing efficiency and versatility in manufacturing.
How Does Thermoplastic Injection Molding Work?
The thermoplastic injection molding process is a symphony of precision and technological innovation, where every stage plays a crucial role in shaping the final product. It begins with the preparation of plastic materials and concludes with the creation of a fully functional part, ready for use.
Material Selection
Material selection is a critical first step in the thermoplastic injection molding process. The choice of material impacts the part’s functionality, aesthetics, and longevity. Commonly used thermoplastic polymers, like polyethylene and polycarbonate, are selected based on their mechanical properties, heat resistance, and suitability for the intended application.
Material Preparation
Preparation involves treating raw plastic pellets for optimal performance. This includes drying to remove moisture, which could affect the melting process and the quality of the molded part. The pellets are then loaded into the injection molding machine’s hopper.
Melting
In the melting stage, plastic pellets are heated in a barrel with a reciprocating screw, turning them into a molten state. Precise temperature control is paramount to achieve the desired viscosity and flow characteristics of the molten plastic.
Injection
During injection, the molten plastic is propelled into a mold cavity under high pressure. This stage is critical for defining the part’s shape and surface finish. The injection pressure and speed are carefully calibrated to fill the mold completely and uniformly.
Cooling and Solidifying
Once injected, the plastic begins to cool and solidify within the mold. Cooling time is essential for the integrity of the part and is influenced by the thermoplastic’s thickness and thermal properties.
Ejection
After cooling, the part is ejected from the mold. Ejector pins facilitate this process, ensuring the part is released without damage. The precise timing and force of ejection are vital to maintain the quality of the finished part.
Post-Processing
Post-processing involves various methods to refine the part, including trimming excess material, polishing for surface finish, and painting if necessary. These techniques enhance the part’s appearance and functionality, meeting the specific requirements of the application.
What Machines are Used for Thermoplastic Molding?
In the realm of thermoplastic injection molding process, specialized machines are the workhorses that bring designs to life. These machines, engineered for precision and durability, play a pivotal role in transforming raw plastic pellets into intricately molded parts. Understanding the types and functionalities of these machines is essential in appreciating the complexities of the thermoplastic molding process.
What Are the Key Components of a Thermoplastic Injection Molding Machine?
A thermoplastic injection molding machine is a marvel of modern engineering, comprising various components that work in unison to produce high-quality plastic parts. Each component is critical in ensuring the efficiency and accuracy of the molding process.
Clamping Unit
The clamping unit of an injection molding machine is responsible for holding the mold halves firmly together during the injection process. It must exert sufficient force to counter the high pressure of molten plastic being injected, ensuring the mold remains closed and the part shape is accurately formed.
Injection Unit
The heart of the machine, the injection unit, is where the plastic material is melted and then injected into the mold cavity. It consists of a heated barrel equipped with a reciprocating screw, which pushes the molten plastic through the nozzle into the mold.
Dwelling and Cooling System
After injection, the dwelling and cooling system plays a crucial role. It maintains pressure on the molten plastic inside the mold, allowing it to fully occupy the cavity and solidify into the desired shape. Efficient cooling is vital to reduce cycle times and enhance part quality.
Ejection Process
Once the plastic part has cooled and solidified, the ejection process comes into play. This involves retracting the mold and using ejector pins to push the finished part out of the mold cavity. The precision and timing of the ejection process are crucial to prevent damage to the part.
Mold Tool
The mold tool, often made of steel or aluminum, is a critical component that defines the shape, surface texture, and size of the final product. It consists of two halves that close together during the injection process. The design and quality of the mold tool are paramount in determining the part’s final characteristics.
What are the Key Parameters in Thermoplastic Injection Molding?
The precision of thermoplastic injection molding hinges on several key parameters, each playing a vital role in determining the quality, efficiency, and overall success of the manufacturing process. Understanding and controlling these parameters is crucial for achieving optimal results in producing high-quality thermoplastic injection molded parts.
Melt Temperature
Melt temperature, typically ranging from 200°C to 300°C depending on the material, is critical for ensuring the plastic flows smoothly into the mold. It directly affects the viscosity of the molten plastic, impacting the ease of filling the mold cavity and the quality of the surface finish.
Mold Temperature
The mold temperature, usually set between 20°C and 120°C, influences the cooling rate and crystallinity of the thermoplastic material. Proper mold temperature ensures faster cycle times and reduces defects like warping or shrinkage in the final part.
Injection Pressure
Injection pressure, often in the range of 500 to 1500 bar, is essential for pushing the molten plastic into every part of the mold. Adequate pressure is necessary for replicating intricate details and achieving a high-quality surface finish.
Holding Pressure
After injection, holding pressure (slightly lower than injection pressure) is applied to compensate for material shrinkage as the plastic cools. This pressure is crucial for maintaining the dimensions and integrity of the molded part.
Injection Speed
Injection speed, which can vary significantly based on part complexity, influences the filling pattern and the appearance of the part. Fast injection speeds are used for thin-walled parts, while slower speeds are better for thicker sections to avoid defects.
Cooling Time
Cooling time, dependent on the thickness of the part and the thermoplastic material used, typically ranges from a few seconds to several minutes. This time is critical for determining the cycle time and the part’s dimensional stability.
Back Pressure
Back pressure (ranging from 5 to 20 bar) is applied to the molten plastic in the barrel, improving color dispersion and removing air from the material. This parameter is essential for material quality and consistency.
Screw Speed
The screw speed in the injection unit, typically between 100 and 200 RPM, affects the quality of the plastic melt. Proper screw speed ensures uniform temperature and mixture of the molten material.
Ejection Speed and Force
Ejection speed and force must be precisely controlled to prevent distortion or damage to the finished part. The ejection system should be strong enough to eject the part efficiently but gentle enough to preserve its integrity.
Residence Time
Residence time, the duration the plastic material stays in the heated state, should be minimized to prevent thermal degradation. This parameter is crucial for maintaining the mechanical properties of the thermoplastic material.
Clamp Force
Clamp force, necessary to keep the mold closed during injection, typically ranges from a few tons to several thousand tons. Adequate clamp force is essential to counteract the high injection pressure and prevent mold flash.
Shot Size
Shot size, referring to the amount of plastic injected into the mold, must be accurately calibrated to fill the mold completely without causing excess material. It is a key factor in determining the weight and volume of the finished part.
Nozzle Temperature
Nozzle temperature, usually similar to the melt temperature, is crucial for maintaining the flow and consistency of the plastic as it enters the mold. Proper nozzle temperature ensures smooth injection and prevents premature cooling of the material.
What are the Different Mold Types and Tooling Used for Thermoplastic Injection Molding?
In thermoplastic injection molding, the choice of mold type is as crucial as the material itself. Each mold type and its corresponding tooling bring distinct advantages, tailored for specific applications.
The diversity of these molds enables manufacturers to produce a vast range of thermoplastic injection molded parts, each meeting unique requirements of strength, precision, and aesthetics.
Two-Plate Molds: The most common and basic mold type in plastic injection molding. These molds consist of two plates that separate at the parting line during ejection. Ideal for simpler parts without undercuts, they offer cost-effectiveness and easy manufacturing.
Three-Plate Molds: These molds have two parting lines and are used for parts requiring more complex gate systems. They allow automatic separation of the part from the runner system, which is beneficial for automation in mass production.
Stack Molds: Stack molds multiply the mold’s output, having several mold parting surfaces, or ‘stacks,’ in the same mold. They are used for high-volume production, effectively doubling or tripling the production capacity with a relatively small increase in machine size.
Tandem Molds: Tandem molds have two molds in one machine that open and close independently. This design allows for molding two different parts or running two different materials in the same cycle, enhancing production flexibility.
Family Molds: These molds produce different parts of a product or different products in one cycle. While cost-effective, the challenge lies in ensuring uniform filling of all cavities, as different parts may require different parameters.
Overmolding: This involves molding a layer of material over a previously molded part. It’s used for adding soft touch surfaces on hard plastics or combining different colors or materials in one part.
Insert Molding: Here, metal or other non-plastic parts are molded into the plastic. Common in manufacturing components with integrated metal parts like threaded fasteners.
Hot Runner Molds: These molds have a heated runner system to keep the plastic molten, reducing waste and cycle times. They are suitable for large-scale production but come with higher maintenance and initial cost.
Cold Runner Molds: In contrast, cold runner molds allow the runner and sprue to cool and solidify with the part. The runner system is then ejected along with the part and is either discarded or recycled.
Unscrewing Molds: Used for parts with threads, these molds have a mechanism to unscrew the part from the mold after injection. They are essential for manufacturing threaded caps and closures.
L-Shaped Molds: Ideal for parts with perpendicular undercuts or projections. They have a sliding mechanism to demold parts that cannot be directly ejected.
Collapsible Core Molds: These molds are used for complex parts with internal undercuts or threads. The core of the mold collapses inward to release the part without damage.
Gas-Assisted Injection Molding Molds: Used for hollow or partially hollow parts, these molds allow gas injection into the molten plastic, creating internal cavities in the part.
In-Mold Decoration and Labeling Molds: These molds allow for embedding labels or decorations into the part during the molding process, resulting in durable and high-quality graphics or textures.
What Materials Are Commonly Used in Thermoplastic Injection Molding?
The versatility of thermoplastic injection molding lies in its ability to work with a wide range of materials, each offering unique properties and benefits.
From durable automotive parts to flexible packaging solutions, the choice of material is pivotal in determining the functionality and quality of the final product.
Here, we explore some of the most commonly used materials in thermoplastic injection molding, their inherent properties, and typical applications.
Acrylonitrile Butadiene Styrene (ABS)
Properties: ABS is celebrated for its toughness, robust impact resistance, and excellent dimensional stability.
Applications: This material is extensively used in automotive components, consumer electronics, and toys. Its ability to withstand mechanical stresses makes it ideal for these applications.
Polyethylene (PE)
Properties: Known for its remarkable flexibility and chemical resistance, PE comes in various densities like High-Density PE and Low-Density PE, offering a range of durability levels.
Applications: Its versatility is evident in products like containers, piping, and everyday household items.
Polycarbonate (PC)
Properties: PC stands out for its high impact strength, transparency, and notable heat resistance.
Applications: It’s widely used in eyewear lenses, bulletproof glass, and sophisticated medical devices, capitalizing on its clarity and durability.
Polypropylene (PP)
Properties: PP is recognized for its chemical resistance, elasticity, and resistance to fatigue.
Applications: This material is often selected for automotive parts, various packaging solutions, and textiles due to its resilience and adaptability.
Polyamide (Nylon)
Properties: Nylon is characterized by its high strength, excellent temperature resistance, and superior wear resistance.
Applications: These properties make it suitable for automotive components, mechanical parts, and various consumer goods that require durability.
Polyvinyl Chloride (PVC)
Properties: PVC’s durability, chemical resistance, and flame retardancy make it a material of choice for numerous applications.
Applications: It’s commonly used in plumbing pipes, medical tubing, and durable outdoor furniture.
Polyethylene Terephthalate (PET)
Properties: PET is valued for its clarity, strength, and safety for food contact.
Applications: It’s the material behind beverage bottles, various packaging solutions, and synthetic fibers.
Acrylic (PMMA)
Properties: Acrylic offers optical clarity, resistance to weathering and UV light.
Applications: These features make it perfect for use in signage, light fixtures, and window panels.
Polystyrene (PS)
Properties: PS is lightweight and cost-effective, offering good clarity.
Applications: Widely used in disposable cutlery, CD cases, and insulation materials, polystyrene is chosen for its ease of molding and affordability.
Thermoplastic Polyurethane (TPU)
Properties: TPU is known for its outstanding elasticity, oil resistance, and abrasion resistance, making it a versatile choice.
Applications:
Footwear: For soles and insoles due to its flexibility and durability.
Medical Devices: Used in flexible tubing and other components requiring elasticity.
Automotive Parts: Ideal for seals, gaskets, and other parts requiring durability and flexibility.
Polyoxymethylene (POM)
Properties: POM is characterized by high stiffness, low friction, and excellent dimensional stability, which are desirable in precision engineering.
Applications:
Precision Parts: Such as gears and bearings in mechanical assemblies.
Electrical Components: Due to its insulating properties.
Consumer Goods: In items like zippers and fasteners requiring strength and precision.
Polybutylene Terephthalate (PBT)
Properties: PBT offers good electrical properties, heat resistance, and chemical resistance.
Applications:
Electrical Components: For connectors and insulators.
Automotive Parts: In under-hood components due to heat resistance.
High Impact Polystyrene (HIPS)
Properties: HIPS is known for its impact resistance and ease of machining.
Applications:
Model Building: For sturdy, yet easy-to-shape models.
Sign Making: Due to its rigidity and durability.
Product Housings: For consumer electronics where impact resistance is key.
Thermoplastic Elastomers (TPE)
Properties: TPE combines the properties of plastics and rubbers, offering flexibility and stretchability.
Applications:
Seals and Gaskets: Where flexibility and a tight seal are necessary.
Soft-Touch Components: In consumer products for ergonomic grips.
Polyphenylene Oxide (PPO)
Properties: PPO is recognized for its high heat resistance, dimensional stability, and electrical insulating properties.
Applications:
Automotive Parts: For components exposed to high temperatures.
Electrical Components: In connectors and housings.
Appliances: For parts requiring durability and heat resistance.
Liquid Crystal Polymer (LCP)
Properties: LCP offers excellent mechanical strength, high temperature tolerance, and good chemical resistance.
Applications:
High-Performance Electrical Connectors: Due to its strength and insulating properties.
Microwave Cookware: As it withstands high temperatures without deforming.
Polyetherimide (PEI)
Properties: PEI is characterized by its high heat resistance, strength, and flame retardancy.
Applications:
Aerospace: For components that require heat resistance and strength.
Medical Applications: In surgical instruments and other heat-resistant devices.
Polyether Ether Ketone (PEEK)
Properties: PEEK is noted for its exceptional high temperature resistance, chemical resistance, and mechanical properties.
Applications:
Aerospace: In components that require durability under extreme conditions.
Automotive: For parts that must withstand high temperatures and stresses.
Medical Implants: Due to its biocompatibility and strength.
Polyphenylene Sulfide (PPS)
Properties: PPS offers high temperature resistance, chemical resistance, and dimensional stability.
Applications:
Automotive Parts: Especially in under-hood applications.
Electrical and Electronic Components: For connectors and circuit boards.
Coatings: Where chemical and heat resistance are necessary.
Styrene Acrylonitrile (SAN)
Properties: SAN is known for its clarity, rigidity, and chemical resistance.
Applications:
Food Containers: As it is clear and resistant to fats and oils.
Kitchenware: For durable, heat-resistant items.
Bathroom Fittings: Due to its resistance to chemicals and cleaning agents.
Acetal (Polyoxymethylene, POM)
Properties: Acetal, known for its high stiffness, low friction, and excellent dimensional stability, is a high-performance engineering thermoplastic.
Applications:
Precision Parts: Ideal for gears, bearings, and mechanical components requiring tight tolerances.
Electrical Insulators: Due to its excellent electrical properties.
Consumer Products: In applications like zippers and window latches where strength and wear resistance are needed.
Ethylene Vinyl Acetate (EVA)
Properties: EVA is celebrated for its flexibility, toughness, and transparency. It’s a rubber-like material that’s also moldable and recyclable.
Applications:
Foam Products: Used in padding and cushioning materials, such as sports equipment.
Footwear: Particularly in soles and insoles for its cushioning properties.
Packaging Films: EVA’s flexibility and toughness make it suitable for various packaging applications.
H3: Polyurethane (PU)
Properties: PU offers a broad spectrum of hardness and flexibility, coupled with durability. It’s a versatile polymer that can be both rigid and elastic.
Applications:
Foam Seating: In furniture and automotive interiors for its comfort and durability.
Wheels and Tires: Particularly in industrial and recreational vehicles.
Automotive Interiors: For dashboard and other interior components.
Polyphenylsulfone (PPSU)
Properties: PPSU stands out for its high heat resistance, toughness, and stability in steam sterilization, making it an ideal material for demanding environments.
Applications:
Medical Instruments: Especially those requiring frequent sterilization.
Aircraft Interiors: For components that need to withstand high temperatures and stress.
Plumbing: In applications where heat resistance and durability are critical.
Polyimide (PI)
Properties: Polyimide is renowned for its exceptional heat resistance, electrical insulation properties, and chemical resistance.
Applications:
High-Temperature Electrical Insulation: In aerospace and industrial applications.
Aerospace Components: For parts exposed to extreme environmental conditions.
Semiconductor Manufacturing: Due to its ability to withstand high temperatures and chemical exposure.
Polyethylene Naphthalate (PEN)
Properties: PEN is similar to PET but offers better barrier properties, heat resistance, and chemical resistance.
Applications:
High-Quality Packaging: Especially where durability and barrier properties are needed.
Electronics: In components that require dimensional stability and insulating properties.
Polybutylene (PB)
Properties: Polybutylene is valued for its flexibility, heat resistance, and pressure resistance.
Applications:
Piping Systems: Suitable for both hot and cold water distribution.
Underfloor Heating: Due to its ability to withstand high temperatures and pressures.
Polymethylpentene (PMP)
Properties: PMP is known for its transparency and heat resistance, making it a unique thermoplastic.
Applications:
Laboratory Equipment: Especially in applications where chemical resistance and clarity are needed.
Microwave Cookware: Due to its ability to withstand high temperatures without affecting food quality.
Polysulfone (PSU)
Properties: PSU is distinguished by its high temperature resistance, strength, and transparency.
Applications:
Medical Devices: Particularly in reusable devices requiring sterilization.
Water Membranes: For filtration systems due to its stability and strength.
Electrical Components: In applications where insulation and heat resistance are critical.
How Do Material Properties Affect the Thermoplastic Molding Process?
The thermoplastic molding process is significantly influenced by the properties of the materials used. Each material brings a unique set of characteristics that dictate how it behaves under the stresses of injection molding, affecting everything from the molding temperature to the final part’s properties.
Melting Point and Heat Resistance: Materials like Polyphenylene Sulfide (PPS) have high melting points and heat resistance, making them suitable for components that face high thermal stress, such as automotive under-hood parts. However, this also means they require higher temperatures during molding, which can impact energy consumption and cycle times.
Viscosity and Flow Rate: The viscosity of a material, like that of Polyethylene (PE), determines its flow rate during injection. Low viscosity materials flow more easily into the mold, ideal for intricate parts, but may require more precise control to prevent defects.
Strength and Flexibility: Materials like Polyurethane (PU) offer a range of hardness and flexibility, allowing for the creation of parts that range from rigid structural components to flexible hinges.
Chemical Resistance: For products exposed to harsh chemicals, materials with high chemical resistance, such as Polyvinyl Chloride (PVC), are essential. This property is crucial for medical devices and piping systems.
Shrinkage and Warping: Different materials shrink at different rates upon cooling. Acetal (POM), for example, has low shrinkage, which is advantageous for precision parts but requires careful mold design to compensate for these characteristics.
Surface Finish and Aesthetics: Materials like Polymethylpentene (PMP) offer clarity and can be used for applications requiring transparency, like laboratory equipment. The material chosen affects the surface finish, which is critical for both aesthetic and functional purposes.
How to Select the Right Thermoplastic Material for Your Project?
Selecting the right thermoplastic material for a project requires a deep understanding of both the material properties and the requirements of the final product. Here are some guidelines and practical tips for material selection:
Understand the Application Requirements: For instance, if the part will be subjected to high temperatures, materials with high heat resistance like Polyphenylene Sulfide (PPS) would be appropriate. For outdoor applications, UV-resistant materials like Acrylic (PMMA) might be necessary.
Consider Mechanical Properties: If the part needs to bear loads or resist impact, materials with high tensile strength and impact resistance, such as Nylon (Polyamide), should be considered.
Evaluate Chemical and Environmental Resistance: In applications like medical devices or outdoor furniture, materials that can withstand sterilization processes or weather conditions, such as Polyvinyl Chloride (PVC) or Polyurethane (PU), are preferable.
Assess Processing Characteristics: Some materials, like Low-Density Polyethylene (LDPE), are easier to mold and may reduce manufacturing costs and complexity. However, they might not be suitable for high-precision or high-strength applications.
Balance Cost and Performance: While some high-performance materials like Polyether Ether Ketone (PEEK) offer exceptional properties, they can be costly. It’s crucial to balance the cost with the performance requirements of the application.
Compliance and Regulations: Ensure the material complies with industry-specific regulations, especially in sectors like food and beverage, medical, and children’s products.
Prototype and Test: Before finalizing a material, create prototypes to test and validate the material’s performance in real-world conditions. This step can prevent costly modifications later in the production process.
What Are the Applications of Thermoplastic Injection Molding?
Thermoplastic injection molding is a versatile manufacturing process used across various industries. Its adaptability to different materials and precision in shaping complex designs make it a preferred method for producing a wide range of products. Here are some of the key applications in different industries:
Automotive Industry:
Dashboard components, bumpers, and car door panels.
Under-the-hood components like fluid reservoirs and housings.
Medical Industry:
Disposable syringes, surgical instruments, and medical device housings.
Components for diagnostic devices and prosthetics.
Consumer Electronics:
Enclosures for smartphones, remote controls, and computer components.
Battery casings and connectors.
Packaging Industry:
Containers, caps, and closures for food and beverages.
Cosmetic packaging and pharmaceutical bottles.
Construction Industry:
Pipe fittings, plumbing components, and electrical boxes.
Insulation materials and window frames.
Toys and Recreation:
Action figures, puzzles, and board game components.
Outdoor equipment like garden tools and playground structures.
Household Products:
Kitchen appliances, containers, and utensils.
Storage bins and cleaning tools.
Is Thermoplastic Injection Molding Expensive?
The cost-effectiveness of thermoplastic injection molding varies based on several factors, including the complexity of the design, the type of material used, and the volume of production. Here’s a general overview:
Cost Per Unit: The average price per unit in thermoplastic injection molding can range widely. For high-volume production, the cost per unit can be relatively low, benefiting from economies of scale. For example, producing a standard plastic gear might cost between $0.10 to $1.00 per unit for high-volume orders.
Mold and Tooling Costs: Initial costs can be significant, often ranging from $1,000 to $50,000, depending on the complexity and size of the mold. However, these costs are amortized over the lifespan of the mold, which can produce thousands or even millions of parts.
Comparison with Other Manufacturing Methods: Compared to methods like CNC machining or 3D printing, thermoplastic injection molding is generally more cost-effective for mass production due to its faster production rates and lower material waste. For instance, while 3D printing may offer lower startup costs for low-volume production, its per-unit cost remains higher compared to injection molding at large scales.
Material Costs: Material costs can vary, with common plastics like polyethylene being more affordable compared to engineering-grade resins. The choice of material impacts the overall cost, with more specialized materials leading to higher expenses.
How Do You Troubleshoot Common Issues in Thermoplastic Injection Molding?
Troubleshooting in thermoplastic injection molding is crucial for maintaining quality and efficiency. Here are common issues encountered during the process, along with best practices for resolving them:
Short Shots: Occurs when the mold isn’t filled completely. This can be resolved by increasing the injection speed or pressure, ensuring the material is at the correct temperature, or adjusting the gate size.
Flash Formation: Excess plastic that escapes from the mold cavity, forming a thin layer on the part’s edge. This is typically addressed by reducing injection pressure, clamping force, or checking the mold for damage.
Warping: Deformation of the part as it cools. To prevent warping, ensure uniform cooling of the part and consider adjusting the mold temperature or cooling time.
Sink Marks: Small depressions or indentations on the surface of the molded part. Increasing the cooling time or adjusting the holding pressure can help minimize sink marks.
Burn Marks: Black or brown discoloration on the part, often due to overheated material or trapped air. Reduce the melt and mold temperature and increase the injection speed to resolve this issue.
Best Practices for Injection Molding:
Regular Maintenance: Keep the injection molding machine and molds in optimal condition through regular maintenance and cleaning.
Optimize Material Use: Select the appropriate material for the part and application. Test different materials to find the best fit for your product’s requirements.
Monitor and Control Process Parameters: Carefully monitor parameters like temperature, pressure, and injection speed, and make adjustments as needed for optimal performance.
Quality Control: Implement rigorous quality control measures to identify and address defects early in the production process.
What is the Difference Between Thermoset and Thermoplastic Injection Molding?
What is the Difference Between Thermoset and Thermoplastic Injection Molding?
Understanding the difference between thermoset and thermoplastic materials is fundamental in the injection molding industry.
Thermoplastic Materials: These materials become moldable upon heating and solidify when cooled. The process is reversible, allowing thermoplastics to be remelted and reshaped. Examples include polyethylene, polypropylene, and nylon. Thermoplastic injection molding is widely used for its versatility and recyclability. It’s ideal for high-volume production of parts that require flexibility, impact resistance, or transparency.
Thermoset Materials: Once heated and molded, thermoset materials undergo a chemical change that is irreversible. They cannot be remelted or remolded. Examples include epoxy, phenolic, and polyester. Thermoset molding is used for products that need to withstand high temperatures, chemical exposure, or electrical insulation. The materials are typically more robust than thermoplastics but lack the flexibility and recyclability.
The key difference lies in the material properties and the reversibility of the molding process. Thermoplastic materials offer flexibility and recyclability, suitable for a wide range of applications, whereas thermoset materials provide enhanced durability and resistance, ideal for specific high-performance applications.
How Does Thermoplastic Injection Molding Compare to Other Molding Techniques?
Thermoplastic injection molding stands distinct among other molding techniques. Let’s compare it with some of these methods:
Blow Molding: Unlike thermoplastic injection molding, which is ideal for creating solid parts, blow molding specializes in hollow objects, like bottles. The thermoplastic injection molding process offers greater precision and complexity in design.
Compression Molding: This technique is used primarily with thermosetting plastics and involves molding material in a heated mold under pressure. Thermoplastic injection molding differs by injecting molten plastic into a mold cavity under high pressure, providing more detail and complexity.
Rotational Molding: Used for large, hollow parts, rotational molding involves rotating the mold during the process. Thermoplastic injection molding is more suitable for high-volume, intricate parts with tight tolerances.
XTJ is a leading OEM Manufacturer that is dedicated to providing one-stop manufacturing solutions from prototype to production. We are proud to be an ISO 9001 certified system quality management company and we are determined to create value in every customer relationship. We do that through collaboration, innovation, process improvements, and exceptional workmanship.