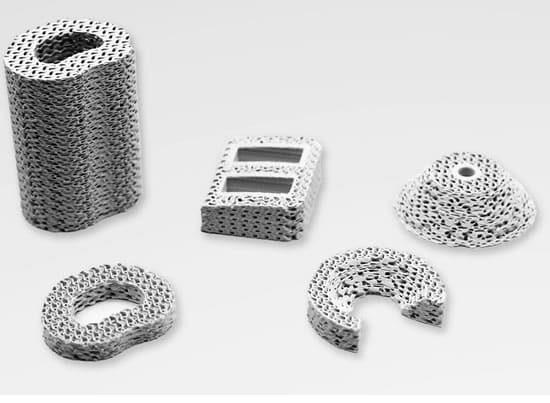
Bioceramics are ceramic materials used in the medical industry to replace or repair hard tissue like bone or teeth. These materials have unique properties that make them very useful for applications like hip replacements and dental implants. Bioceramics also fall into three distinct categories: bioinert, bioactive, or bioresorbable.
This article explores the characteristics, manufacturing processes, materials, and types of bioceramics.
What Are Bioceramics?
Bioceramics can be defined as biocompatible ceramic materials meant to repair parts of the human body, usually to replace hard tissue such as bone and teeth. They include bioinert materials like alumina and zirconia, bioactive materials like hydroxyapatite and bioglass, and resorbable materials such as tricalcium phosphate. Bioceramics are used in many applications, including bone grafting, as components of joint replacements and as dental replacements.
What Is the Bioceramics Production Process?
The production of bioceramics starts with powder synthesis, usually via chemical routes (wet chemical synthesis) like sol-gel or co-precipitation methods to create fine ceramic powders. These powders are then shaped into a preliminary form (green body) using one of the following techniques:
Uniaxial Pressing: Powder is placed in a die and compressed under single-axis pressure to form a green body (an unsintered ceramic piece).
Isostatic Pressing (Cold Isostatic Pressing – CIP): Powder is enclosed in a flexible mold and subjected to isostatic pressure applied uniformly in all directions using a fluid medium, often leading to more uniform density distributions.
The shaping step is followed by sintering, in which the green body is heated to high temperatures that begin to bond the particles together. In conventional sintering, the green body is heated to a temperature below its melting point but high enough to enable diffusion processes that lead to densification and grain growth. This process bonds the particles together and eliminates porosity, enhancing the material’s strength and integrity. Another technique used in ceramic manufacturing is hot pressing which combines pressure and heat in a single step. The ceramic powder is placed in a mold and heated while pressure is applied, accelerating densification and allowing for the fabrication of parts with complex geometries. Hot isostatic pressing (HIP) is a similar method but employs isostatic pressure applied by an inert gas (typically argon) in a high-pressure vessel. This process achieves high density and homogeneity.
Another method for shaping ceramics is 3D printing technology. In particular, selective laser sintering (SLS) and stereolithography (SLA) are good for bioceramics. In SLS, a laser selectively sinters powdered material, bonding it together to form a solid structure. In SLA, a laser will polymerize a liquid resin that contains ceramic particles. Both methods build the item up layer by layer, based on a 3D model. The resulting structure is then subjected to post-processing, including debinding and sintering, to finalize the ceramic part.
After sintering, bioceramic parts often require machining and finishing to meet exacting dimensions and surface quality. Techniques include precision grinding, polishing, and sometimes, coating with specific substances (e.g., hydroxyapatite) to enhance bioactivity or biocompatibility.
What Are the Fundamental Properties of Bioceramics?
The fundamental properties of bioceramics are as follows:
1. Biocompatibility
Bioceramics are designed to be compatible with biological tissues, causing minimal or no adverse reactions when implanted in the body. This property means they can be safely used for medical implants and devices. Biocompatibility stems from the fact that the constituent materials are antibacterial and chemically inert.
2. Mechanical Properties
Bioceramics are characterized by their unique mechanical properties, including low tensile strength, high hardness, and minimal plasticity. This all stems from their strong ionic or covalent bonds. These materials are brittle, making them prone to fractures under low energy impacts due to microscopic imperfections such as pores and micro-cracks. This brittleness leads to poor fatigue resistance as they fracture before showing any plastic deformation. It poses design challenges, especially in load-bearing implants like hip replacements, necessitating careful consideration of shape and material thickness to prevent failure. However, their wear resistance is beneficial for dental implants and bone substitutes.
Despite the challenges, bioceramics possess higher hardness and elastic modulus values compared to metals, making them suitable when wear resistance is the most critical factor. However, their fracture toughness is significantly lower than that of metals, limiting their resistance to crack propagation. Still, their high specific elasticity makes them valuable for reinforcing composite materials and for use in high-temperature environments. Despite the challenges posed by their brittleness, ongoing advancements in bioceramic materials aim to enhance their mechanical performance, broadening their applicability in healthcare.
3. Corrosion Resistance
Bioceramics are chemically inert and resist degradation in the harsh biochemical environment of the human body. This corrosion resistance contributes to their durability and longevity as implants.
4. Osteoconductivity
Some bioceramics, such as hydroxyapatite and certain bioactive glasses, support bone growth on their surfaces. This property is crucial for the success of bone grafts and orthopedic implants.
5. Chemical Stability
Bioceramics do not undergo significant chemical changes in the body. This stability ensures that they do not release harmful substances that could trigger adverse biological responses, such as inflammation or rejection.
6. Thermal Properties
The thermal properties of bioceramics are ideal for use in the human body. Their temperature resistance and relatively low thermal conductivity ensure minimal heat transfer to surrounding tissues, preventing thermal shock and potential damage. Additionally, the close match of their thermal expansion coefficients with those of human tissues minimizes stress at the interface, ensuring the stability of implants during temperature fluctuations. These thermal characteristics, along with their thermal stability, contribute to their value in implants and prostheses.
7. Electrical Properties
Bioceramics, by design, are primarily focused on biological compatibility and integration with body tissues rather than electrical properties. The most common types of bioceramics, such as hydroxyapatite and bioactive glasses, are electrical insulators. Electrical properties are not deciding factors in the design of: bone repair implants, dental implants, and other prosthetic devices. However, some bioceramics can exhibit specific electrical behaviors under certain conditions. For instance, barium titanate has piezoelectric effects when used in bone repair, potentially aiding in bone growth through electrical stimulation. The effect is not as pronounced as those of piezoelectric or ferroelectric ceramics, which are specifically designed for applications in: sensors, actuators, and other electronic devices.
8. Surface Porosity
The porosity of bioceramics can be engineered to promote tissue ingrowth and vascularization, enhancing the integration of implants with host tissues. Porous structures are especially important in bone grafting and in scaffolds for tissue engineering.
9. Radiopacity
Bioceramics are often radiopaque, meaning they are visible to X-ray equipment. This property is required for dental applications and joint replacements.
How Are Bioceramics Used?
Bioceramics are extensively used in the medical field due to their biocompatibility. They play a crucial role in repairing and reconstructing damaged parts of the human body, such as bones and teeth. Applications include: bone grafts, dental implants, and joint replacements. Bioceramics like hydroxyapatite and bioglass support bone growth and integration with the body’s natural tissues, making them invaluable in: orthopedics, dentistry, and tissue engineering.
What Industries Use Bioceramics?
Bioceramics are used predominantly in healthcare, including orthopedics (bone grafts and joint replacements), dentistry (implants and reconstructive surgery), and biomedical devices. Additionally, one of the emerging trends in tissue engineering involves the use of scaffolds that give growing or healing cells a properly shaped form to latch onto. These scaffolds are engineered to biodegrade after fulfilling their purpose, allowing them to be naturally excreted from the body.
What Materials Are Used for Bioceramics?
There is a wide range of materials that can go into bioceramics. Some of these include:
1. Bioactive Glass
Bioactive glasses are silica-based materials that, upon implantation in the body, can bond to bone and stimulate bone growth. These materials release ions that are believed to stimulate cellular responses, leading to the formation of a bond between the implant and bone. They are used in a variety of applications, including bone grafting, as coatings on metal implants to enhance bioactivity, and in the treatment of osteoporosis.
2. Bioglass
Bioglass is a specific type of bioactive glass with a defined composition patented by Larry Hench in the late 1960s. It was the first bioactive glass to be developed and has set the standard for many of the bioactive glasses used today. Bioglass is primarily composed of: silica, calcium oxide, sodium oxide, and phosphorus oxide. It is used for similar applications as bioactive glass, promoting bone bonding and regeneration.
3. Calcium Phosphate Ceramics
Calcium phosphate ceramics closely resemble the mineral composition of bone, making them highly biocompatible. They include hydroxyapatite and various forms of tricalcium phosphate. These materials are used for bone grafts, dental implants, and as coatings on metal implants to promote osteointegration.
4. Alumina
Alumina (aluminum oxide, Al2O3) is a ceramic known for its hardness, wear resistance, and biocompatibility. It is used in: load-bearing orthopedic implants, dental implants, and as components in hip and knee replacements.
5. Calcium Sulfate
Calcium sulfate, also known as gypsum, is a resorbable biomaterial used in bone regeneration and as a bone graft substitute. It acts as a scaffold, supporting new bone growth, and gets gradually replaced by natural bone over time. Calcium sulfate is particularly useful for filling bone defects and promoting bone healing.
6. Silicon Nitride
Silicon nitride (Si3N4) is a ceramic material known for its excellent mechanical properties, including its: strength, toughness, and wear resistance. It also exhibits good biocompatibility and antibacterial properties, making it suitable for: spinal implants, dental implants, and other orthopedic applications.
7. Zirconia
Zirconia (zirconium oxide, ZrO2) is a ceramic material with notable fracture toughness and excellent wear resistance. It is used in dental and orthopedic implants because it looks like natural tooth enamel and is similarly strong. Zirconia can withstand high stress and is suitable for hip and knee replacements, dental crowns, and dental bridges.
8. Tricalcium Phosphate
Tricalcium phosphate (TCP, Ca3(PO4)2) is used as a bone graft substitute and also shows up in tissue engineering. TCP is bioresorbable and supports bone ingrowth, making it an effective scaffold for bone regeneration. It is available in various forms, including β-TCP, which has a different crystal structure.
9. Hydroxyapatite
Hydroxyapatite (HA, Ca10(PO4)6(OH)2) is a naturally occurring mineral form of calcium apatite and is the primary component of bone mineral and tooth enamel. Synthetic hydroxyapatite is widely used in orthopedics and dentistry for bone grafts, as a coating on metal implants to promote bone growth and integration, and in dental implants and fillings.
The ideal choice of material depends on the required mechanical properties, biocompatibility, bioactivity, and the specific clinical application. Materials that can’t be used include: heavy metals (e.g., lead, mercury) due to toxicity, non-bioresorbable polymers with poor biocompatibility that can provoke immune responses, and non-inert metals prone to corrosion or allergic reactions. Brittle materials with low mechanical strength must also be avoided, along with ceramics that dissolve too quickly in bodily fluids, potentially releasing harmful substances. Additionally, materials with high thermal conductivity that can cause thermal damage to tissues and specific glass compositions that leach toxic elements are not used in bioceramics due to their adverse interactions with biological systems.
What Machines Are Used to Produce Bioceramics?
Several specialized devices are used throughout the manufacturing process for bioceramics. These machines represent various stages of production, from powder synthesis to shaping, sintering, and finishing. Here’s a brief overview of machines used in the production of bioceramics:
Ball Mills: Ball mills are used for grinding and milling. They work by rotating a cylinder with grinding media, like ceramic balls, causing the balls to repeatedly fall back into the cylinder and onto the material to be ground. This process is essential for creating fine, homogeneous ceramic powders.
Spray Dryers: Spray dryers use hot gas to quickly dry slurry or liquid feed into dry powder. This method is beneficial for preparing powders with controlled particle sizes and porosities so they’ll be ready for pressing and sintering processes.
Presses: These presses compact ceramic powders into desired shapes. Hydraulic presses apply uniform pressure, which is essential for achieving consistent density throughout the ceramic part. Mechanical presses are used for uniaxial pressing, forming powders into green bodies ready for sintering.
Cold Isostatic Presses (CIP): Cold isostatic pressing encases the ceramic powder in a flexible mold that’s then subjected to isostatic pressure applied by a liquid medium. CIP is used to achieve uniform densities and eliminate material defects.
Sintering Furnaces: Sintering furnaces bring the ceramic parts to the proper density and mechanical strength. These furnaces must provide precise temperature control and atmosphere conditions to ensure the integrity of the bioceramics.
Hot Isostatic Pressing (HIP) Machines: HIP machines apply high temperature and isostatic gas pressure (often argon) to densify ceramics in all directions simultaneously. This method is particularly useful for removing residual porosity.
3D Printers for Additive Manufacturing: Stereolithography (SLA), selective laser sintering (SLS), and other 3D printing technologies are increasingly common ways to produce bioceramic parts with complex geometries directly from computer models. These machines layer material bit by bit to form structures that can closely mimic the natural architecture of human tissues.
What Are Examples of Bioceramics?
Here are some common examples of bioceramics:
Alumina (Aluminum Oxide) for orthopedic joint replacements and dental implants
Zirconia (Zirconium Oxide) for dental crowns, bridges, and implants
Hydroxyapatite (HA for bone repair and reconstruction.)
Bioglass for bone grafts, dental reconstruction, and as a coating to promote bonding between implants and bone
Calcium Phosphates for bone repair and augmentation
Silica-Based Ceramics for bone grafting and as coatings on implants to enhance integration with biological tissues
What Are the Different Types of Bioceramics?
Bioceramics can be classified into three main types based on their interaction with the human body. These categories help differentiate their applications based on how they function when implanted or how they’re used in medical procedures:
Bioinert Ceramics: Bioinert ceramics, such as alumina (aluminum oxide) and zirconia (zirconium oxide), are characterized by their lack of interactions with biological tissues. These materials are chosen for their stability and resistance to wear and degradation within the body, maintaining their structure and functionality over time without eliciting any adverse biological response. They are primarily used in joint replacements and dental implants. Their exceptional mechanical strength and wear resistance make them ideal candidates for the harsh mechanical rigors of orthopedic implants.
Bioactive Ceramics: Bioactive ceramics, including hydroxyapatite (HA) and Bioglass, are designed to interact positively with biological tissues — particularly bone. These materials can bond directly with the bone, facilitating the natural healing process and encouraging the integration of implants with the surrounding skeletal structure. Their chemical composition is similar to that of the natural bone mineral, making them highly biocompatible and effective in supporting bone growth and regeneration. This property is exploited in a wide range of applications, from bone grafting materials and coatings that enhance osteointegration to dental implants that require a strong bond with jawbone tissue.
Resorbable (Biodegradable) Ceramics: Resorbable ceramics, such as tricalcium phosphate (TCP), are designed to gradually degrade and be replaced by natural bone tissue over time. This category of bioceramics is particularly beneficial when the implant should be temporary. It provides support or stimulation for natural bone growth and then gets safely absorbed by the body. The controlled resorption rate of these materials allows the ceramic to be naturally replaced with newly formed bone, making them ideal for bone repair and augmentation procedures. This biodegradability minimizes the need for additional surgeries to remove the implant, offering a more natural and less invasive approach to bone regeneration.
Are Bioceramics Tailored To Have Mechanical Properties Similar to Natural Bone?
Yes, some bioceramics are indeed tailored to have mechanical properties similar to natural bone. The aim is to match the stiffness, strength, and toughness of the surrounding bone tissue to ensure that the implanted material integrates well without causing stress shielding or bone resorption. Bioceramics like hydroxyapatite and bioactive glasses are designed to closely mimic the mineral component of bone, promoting bone growth and bonding to the surrounding tissue. Researchers can tailor their mechanical properties to closely match those of the bone by adjusting the composition, porosity, and structure of these materials. This enhances their effectiveness in: bone grafts, implants, and other orthopedic applications.
What Is the Difference Between Bioceramics and Piezoelectric Ceramics?
Bioceramics and piezoelectric ceramics serve different purposes and possess unique properties. The primary difference lies in their functional purpose: bioceramics are engineered for biological interaction and integration, while piezoelectric ceramics change electrical behavior in response to mechanical stresses. Piezoelectrics are not intended for implantation or direct biological applications.
XTJ is a leading OEM Manufacturer that is dedicated to providing one-stop manufacturing solutions of Machining 6061 Aluminum from prototype to production. We are proud to be an ISO 9001 certified system quality management company and we are determined to create value in every customer relationship. We do that through collaboration, innovation, process improvements, and exceptional workmanship.lication: Automotive industry, Bicycle and motorcycle, Door and windows and furniture, Household appliance, Gas meter, Power tool,LED lighting, Medical instrument parts, ect.